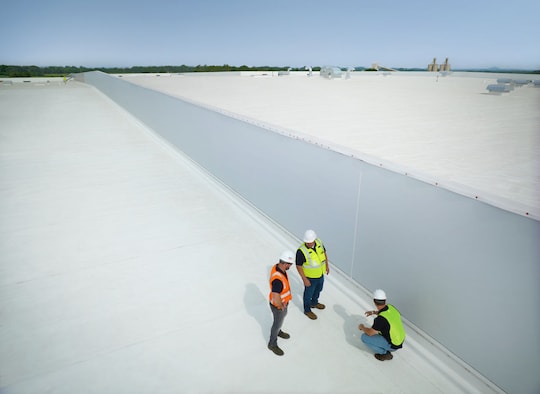
The high output from bifacial modules could be extended for many years using a long-life membrane.
Unlike conventional solar panels, bifacial panels collect light from both sides — top and bottom. When mounted above highly reflective surfaces, their efficiency can exceed that of regular modules. Due to this benefit, bifacial panels are becoming more readily available. Estimates of the amount of increased power bifacial panels provide range between 20% and 35%. Clearly, this depends on the amount of light that each side receives. Therefore, there are implications for membrane specification when such modules are installed on low-slope roofs. The use of bifacial solar panels is growing fast, making it worthwhile to explore the topic in depth.
To understand the importance of membrane choice, it is useful to briefly review solar panel design. Conventional solar panels rely on a reflective back surface to improve efficiency as shown in this schematic:
Solar cells are not highly efficient and some of the sun's energy passes through them. By reflecting that energy back up through the cell, the probability of converting it to electrical energy is increased. The back reflective layer serves double duty, consisting of aluminum it also acts as a collector for the power being produced.
Bifacial solar panels do away with a reflective continuous metal back film and demand a more sophisticated cell design to ensure that the electrical energy is harvested efficiently. They are able to absorb solar energy from both sides with the general concept being as shown here:
Bifacial panels are not equally efficient in each direction and the rear direction usually operates at about 75% of what is achieved from the front. Clearly, such panels should be installed above highly reflective surfaces, such as cool roof membranes, as shown here:
The overall efficiency of bifacial modules is dependent upon the amount of light that reaches the rear side. LG,* a manufacturer of bifacial solar panels, has modeled how much additional power one of their panels could produce depending on the surface underneath. The following graphic from LG suggests that a white membrane could increase power output by 26.9%.
Cool roof membranes are known as diffuse reflectors as compared to mirrors which are specular reflectors. The difference is shown here:
The diffuse reflectance from a roof membrane has the potential to illuminate the underside of a bifacial solar module and drive output up significantly.
In practice, these bifacial panels are easily identified by the absence of a plastic back layer which is replaced with a glass layer, as shown in this example:
Source: Opsun Systems Inc.
There are many manufacturers of bifacial solar panels, including Canadian Solar, Jinko Solar, LG, LONGi, Sunpreme, Trina Solar, and Yingli Solar.* To enable light to enter from both sides, bifacial solar panels have glass on both faces, and therefore panel life may be longer. Additionally, bifacial panels can be produced without an aluminum frame, which may partly offset the cost of the extra sheet of glass.
As stated earlier, the output of bifacial solar panels is dependent on the reflectivity of the substrate. In the case of TPO single-ply roofing membranes, there are generally only minor differences between TPO membranes from different manufacturers in terms of initial reflectance. The critical measure is solar reflectance since it is visible light that provides energy for conversion to electricity. Solar Reflectance Index, or SRI, is not appropriate because it includes an emittance term which is a measure of heat being radiated from the surface.
The independent Cool Roof Rating Council shows GAF EverGuard® TPO to have an initial reflectivity of 0.76, in line with other standard TPO membranes. The three-year aged reflectivity is shown as 0.68, again in line with other TPO membranes. However, GAF EverGuard Extreme® has an initial reflectivity of 0.83, i.e., 7 percentage points higher than the standard TPO. The three-year aged value is stated to be 0.72.
The three-year aged membrane values are frequently regarded as being representative of "long term" reflectivity. However, this relationship is based on standardized testing and does not take into account site-specific issues or differences between membrane types. Membrane dirt pick-up is determined by many factors including location, roof slope, and the chemistry of the membrane. Discussion of location is beyond the scope of this article, although it is likely that a roof in an industrialized area could get dirtier than a similar roof in a rural area. There is significant anecdotal evidence that single-ply roofs without standing water stay cleaner than those with poor drainage. Single-ply assemblies with tapered polyiso, enabling good drainage, likely stay cleaner longer than roofs with limited to no slope and poor drainage, all other things being equal.
In terms of single-ply membrane type, PVC contains liquid plasticizers that migrate to the surface, which may make the membrane feel slightly sticky. Depending on the exact formulation, PVC can become gray with dirt that does not readily wash off.
As compared to PVC, TPO membranes are unlikely to exhibit stickiness or tackiness. Long-term dirt retention of TPO membranes depends on the type of dirt (e.g., dust versus industrial contaminants) and the smoothness of the membrane surface. By accelerating the aging using the same UV exposure test as in the ASTM D6878 TPO Specification, it is possible to get an indication of changes in surface morphology. The following pictures, taken with a 100X microscope, show the surface of standard TPO compared to that of GAF EverGuard Extreme® after exposure to over four times the ASTM D6878 requirement.
The standard TPO developed a rougher surface compared to that of GAF EverGuard Extreme®, the latter formulated for outstanding UV and heat resistance. This would suggest that the dirt retention of GAF EverGuard Extreme® might be lower than standard TPO and therefore lead to higher output when used in conjunction with bifacial solar panels. It is worth noting that the D6878 specification calls for an inspection of the surface with a 7X eyepiece, while the pictures above were taken using 100X magnification.
At the GAF Cedar City, Utah, facility, EverGuard Extreme® membrane has been installed on a lower roof in front of the building. As shown in the following photographs taken at mid-day during the summer over the past five years, the amount of reflection up onto the adjacent wall is still visibly noticeable.
The pictures help demonstrate the sun's energy that would be available via reflection to bifacial solar panels had any been installed. As noted by NREL, shown in the following figure, there are many variables to be considered when mounting bifacial solar panels:
However, given that annual power output gains of around 30 'Äì 35% have been demonstrated when bifacial solar panels were installed over substrates with a solar reflectance of 0.5 'Äì 0.6, then a membrane such as GAF EverGuard Extreme®, with a potential to maintain high reflectance over a long time period, could provide a major advantage.
In summary, bifacial solar panels can potentially increase the output relative to a traditional solar array by upwards of 30%. It makes sense to protect those gains over the long term by choosing a membrane designed for long life and that has the potential to better resist dirt retention during that time. The right choice of membrane is important for any solar installation, but for bifacial panels it becomes even more so.
*Trade and company names or company products referred to herein are intended only to describe the materials and products discussed. In no case do these references imply recommendation or endorsement, nor does it imply that the particular products are the best available for the purpose discussed.